Climate change, and the solutions needed to mitigate it, represent an enormous challenge - its cause and effect permeate every facet of society. In part 1 we discussed what a bad climate outcome means for society, where greenhouse gas emissions are coming from, limitations we’ll need to keep in mind when discussing solutions, and the scale needed to make a meaningful dent in our emissions.
Part 2 of our ongoing discussion is broken into multiple articles, each one covering one of the five emissions categories. We’ll talk about the solutions that need to be deployed in order to shift our society to one that can utilize energy and resources without emitting greenhouse gases. We’ll primarily be focusing on the physical systems that result in fewer emissions - infrastructure. This change in infrastructure will bring about a whole host of systems and software to facilitate and optimize this shift, which we’ll cover in later sections.
After covering each of the five emissions categories, we’ll discuss how we can suck carbon out of the atmosphere, how we can mitigate the destruction that will come from the climate change already underway, as well as the big role that government-led national and international goals and incentives play.
Innovation Takes Time
Especially when the infrastructure manifests in atoms rather than bits. Software’s nature allows for quick iteration cycles and easy deployment worldwide. Many of the solutions that will overhaul our energy and resource infrastructure require new scientific understanding, high capital expenditures, long iteration cycles, and a lot of energy to scale.
While the past few decades and the recent venture capital interest in clean energy have seen a lot of excitement around the power of software, there’s no way of avoiding the fact that when it comes to how we utilize energy and resources, infrastructure needs to be built and deployed on a massive scale.
As we discussed in part 1, the cost of that technology will go down over time through interaction cycles. However, in the realm of physical infrastructure, costs may be higher for a longer period of time than ideal. When discussing climate solutions, it’s important to know where technologies fall on that development and deployment curve.
To convey this, I’ll highlight where a given solution a) is an obvious, economic or near economic, solution that simply needs to be scaled up b) exists today but needs iteration cycles to bring costs down, and c) requires scientific or engineering breakthroughs to be feasible.
A quick note, which will be discussed more in the section on government’s role: potential solutions in group c) as well as many in b) are not yet economically sustainable, meaning private markets will not be as willing to invest in them as the challenge calls for; this is where government incentives and investment can make a really big impact in helping bring new technologies to market.
This is what happened with early government investment in computers, the internet, GPS, and the Human Genome Project, which all had large societal returns on investment. Once technologies are in group a), markets are a great vehicle to bring these technologies to the world, as long as entrenched interests and regulatory capture do not get in the way.
Emissions Categories
As a reminder, here is where our global emissions come from2:
There are thousands of possible solutions targeting various sources of emissions, with their own risks, costs, and benefits. We’ll be covering some promising solutions over the next few articles, and along the way, I’ll share some resources highlighting more solutions. In this article, we’ll be covering how to bring electricity generation down to net-zero emissions.
Electricity Generation - 27% of emissions
Today, 29% of our energy is generated from renewables, and 10% is generated from nuclear, meaning ~60% of our electricity is generated from fossil fuels worldwide!3 The electricity cycle can be broken down into multiple stages - generation, storage, transmission, usage. Let’s look at these stages and some of their potential solutions.
Generation
Solar, Onshore Wind, Offshore Wind: Solar and wind are now cheaper than most other forms of energy. Wind and solar make up less than 10% of global electricity generation but that number is growing. We are now at a stage where these projects need to be scaled up. Today, most renewable electricity is generated with hydropower, at about 15% globally, though cost reductions in solar and wind make them cheaper solutions where the environment permits. As a result that balance is shifting.4
While scaling up these renewable sources is one of the biggest levers we have, they will not solve all of our problems, as their variability and dependence on weather means we need other systems to ensure we have adequate and predictable electricity generation. Because of the scale of the problem and the different environments in which supply and demand of energy will be balanced, we will need a diverse range of energy sources and storage solutions to bring down the 27% of emissions caused by electricity generation.
Nuclear fission: This is the nuclear we all know and (many don’t) love. It’s controversial, with one legitimate but workable concern being the question of how we dispose of nuclear waste. Another misplaced concern is about safety, a fear that has been amplified by the vivid imagery of Chernobyl and Three Mile Island. Counter to instinct, nuclear energy is actually one of the safest forms of energy generation by far - more people die from coal pollution in a single year than have died in all nuclear accidents combined.5
One of its main benefits is that nuclear doesn’t have intermittency issues (it can run 24/7) and is not reliant on atmospheric and geological conditions. Nuclear energy is expensive today (~9.3¢ compared to ~2-3¢ per kilowatt-hour for solar and wind)6, especially in the US where regulatory inefficiencies make the process expensive and time-intensive.
New “Generation IV” plants are being designed to be cheaper and even safer. One such company focusing on new generation nuclear is TerraPower; while their designs currently live in software simulations, in late 2020 TerraPower received a grant and authorization from the U.S. Department of Energy to build two demonstration plants, expected to be operational in five to seven years.7
Nuclear fusion: It’s ambitious but physically possible, and it would be a holy grail of energy if we crack it. Companies like Commonwealth Fusion and researchers at MIT are working on it, and say it will be here in 15 years.8 Unfortunately, the decades-old industry joke for fusion is: it’s 20 years away and always will be. Hopefully, it truly is 15 years away, but we cannot risk waiting that long to find out.
Geothermal: We are getting to a point where geothermal can begin to scale and companies like Fervo Energy are hard at work to make that happen. The heat energy generated from geothermal is about 100 billion times more than current world energy consumption.9 The problem is that energy is hard to access. The cost of deep tunneling is still prohibitive in many instances and the tools for identifying where to drill are not advanced enough to make finding locations simple. While there is a lot of promise, current technologies leave large-scale geothermal projects economical only in certain locations today.
A quick reference to the cost of various energy sources:
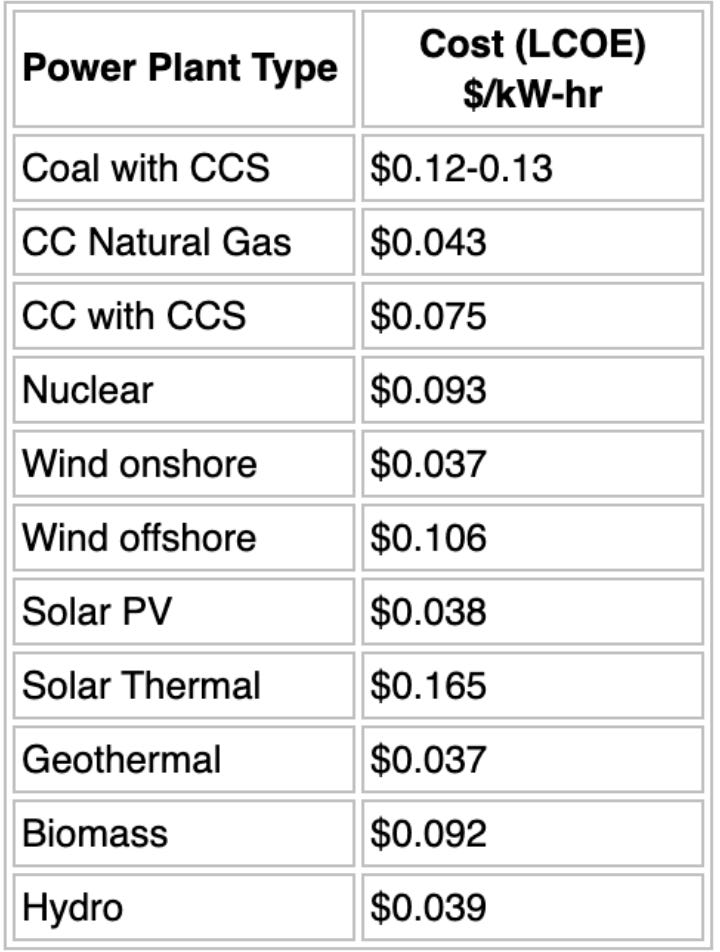
Grid-scale electricity storage
Solar and wind are now extremely cost-effective, but they have the problem of intermittency - if the sun is not shining or the wind is not blowing, how do we power our lives? While battery storage is rapidly improving, it still has a long way to go. For context, the best lithium-ion battery available today packs 35 times less energy than gasoline, pound for pound.11 While renewables generate energy at less cost than fossil fuels, when large-scale storage is factored in, the picture does not look as good.
Let’s look at some levelized costs of energy, which represent the total cost to build and operate a piece of infrastructure over its lifetime divided by the amount of energy it produces or stores.
Diesel, coal, and combined gas cost about 10¢ 6¢ 4¢ per kWh, respectively.
Wind and solar float in the 2¢-3¢ per kWh range, making generation cheaper than fossil fuels. However, higher costs come when we need to store that energy.
Lithium-ion batteries and pumped hydro storage cost about 25¢ and 17¢ per kWh!12 If you need storage with your solar, you’ll need to pay 19¢-27¢ per kWh.
If we want to get to a place where all energy is clean, we need innovation to bring these costs down. Let’s look at different options to get us there, as well as some that probably won’t.
Lithium-ion batteries are the best electrochemical batteries out there when balancing the tradeoffs of capacity (how much energy can be stored) and energy density (how much mass and/or volume is needed to store that energy).13 There are various ways to construct lithium-ion batteries being explored today to reduce costs, increase capacity, increase density (particularly volumetric density for EVs), and optimize manufacturing capabilities. QuantumScape recently rolled out a new solid-state lithium-ion battery (using a solid electrolyte instead of a liquid one), which allows for greater energy density and faster charging.14 While they have not yet scaled up manufacturing, the technology is promising.
Manufacturing and scaling are some of the hardest parts of battery production, and aside from process, there are supply chain constraints to consider. For example, nickel demand is expected to outstrip nickel supply, which is a major element typically used in Li-Ion batteries (there’s traditionally more nickel used than lithium); as a result, Tesla has announced they will be switching from nickel to iron for their standard range cars.15
Tesla, as well as companies like Panasonic and Samsung, are investing heavily to ramp up and optimize manufacturing capabilities for their batteries. There’s too much happening in lithium-ion batteries to cover in this high-level overview, though it’s safe to say we’ll continue to see a reduction in lithium-ion batteries as companies aim to lower prices, increase performance, and scale up manufacturing.
While cost reductions are happening for lithium-ion batteries, there are other forms of energy storage that will play an important role in grid-scale storage, such as mechanical and thermal forms of energy storage.
Hydro storage pumps water up a hill and then allows the water to fall back down the hill, turning potential energy into kinetic energy, and then to electrical energy. This form of energy storage is quite mature and very effective at storing large amounts of energy, representing a surprising 95% of the world’s large-scale electricity storage as of 2016.16
While it’s amongst the cheapest forms of large scale energy storage today, it likely won’t play a major role in energy storage in the future, as a topographical incline is needed, there isn’t that much innovation that can be done to reduce costs, and the current costs just aren’t worth it (reminder, it costs 17¢ per kWh, which is expensive). That is why 90% of new large-scale battery storage being developed is lithium-ion as of 2017.17
There are other forms of mechanical storage that are promising, such as stacking concrete blocks and letting them fall from the top of the stack, transferring potential energy to kinetic energy when needed. One company pioneering in this space is Energy Vault, and they have demonstrations showing they can store energy at 3.5¢ per kW-hour, which would make renewable energy generation + large scale storage cost-competitive with fossil fuels!18 It will be some time before this is a mature and time-tested technology, but innovations like this are promising.
On the thermal storage front, molten salt can be heated by mirrors and stored as thermal energy to be used throughout the day. While this technology has traditionally been expensive, new innovations like those from Heliogen have utilized computer vision to optimize the angle of the mirror based on where the sun is to concentrate solar energy to an extremely dense area. This breakthrough brings thermal storage to a competitive range; it also opens up opportunities because the energy is so concentrated. This heat can be used for industrial processes such as creating steel and cement, which typically use fossil fuels to reach the high temperatures necessary in the smelting process. It also can be used for electricity and hydrogen generation (more on hydrogen in a future article).19
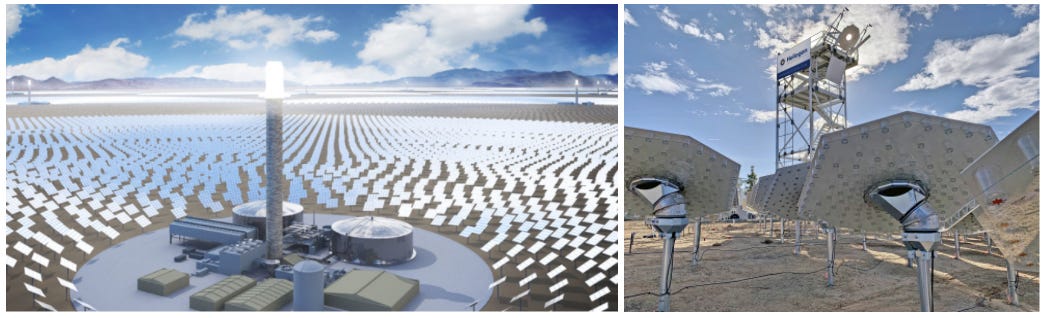
Transmission and Tying it All Together
Modernizing the grid: Parts of the grid (particularly in the US) are about 100 years old and in need of serious repair. The North American Grid is the “world’s largest machine” and was designed and built at a time when utilities were the main generators of energy, which would provide highly predictable patterns of energy supply.
As our grid needs to handle more intermittent sources from diverse places, there will be many challenges as we try to fit new use cases onto an old design. Countless infrastructural changes will need to happen, as well as the creation of new routing systems to ensure our grid supply, demand, and storage is balanced and resilient.
If the grid fails, as it did in Texas recently, our efforts to transition to renewables will be delayed and costs will be higher. Unfortunately, events like the deep freeze in Texas are expected to increase as our old grid infrastructure becomes further obsolete and climate change causes more destructive weather events.
The technologies to make the required changes to the grid – both hardware and software – exist today. The main roadblock will be getting the political will to make the transition at all levels of government, as much of the grid is mired with bureaucratic, financial, and political entrenchment, which I will cover in later sections.
A Promising Future for Electricity
While it will be no easy feat, there is a visible and feasible pathway to net-zero emissions for electricity generation. Getting our electricity generation, storage, and transmission to net-zero at a competitive cost as soon as possible will have broad-ranging implications, as that energy will be used in all parts of our economy: how we make things, how we grow things, how we transport things, and how we warm things.
We have a long way to go, with about 60% of electricity generation coming from fossil fuels, but there is major progress underway: 80% of all new electricity capacity in 2020 was renewable!20
For countries like India and those in Africa, which are seeing rapid development and will be the main source of global energy growth in the coming decades, it will be harder to go fully renewable, as they don’t have the extra wealth to spend on new expensive storage technology. With that being the case, the competitive price of solar and wind energy, and their localized nature, are enabling remote communities to generate electricity in a way that could only be done through fossil-fuel driven projects in the past, which need robust supply chains for ongoing fossil fuels and generally require large scale infrastructure. Just as many developing countries skipped over landlines and went directly to mobile, we may start seeing more towns and cities leapfrog over large-scale national grids and go directly to clean localized microgrids. In off-grid places today, many people are paying four to five times the average price paid for electricity in urban areas, while some are paying up to forty-five times the average electricity price in the US!21 At those prices, installing a modern solar plus storage system will bring huge economic benefits to the community.
As more developers materialize globally, the development process becomes more standardized, business model innovations make costs more manageable (more on this in a future article), and these assets become more financialized, the opportunity for international investment will grow, further accelerating progress.
As we’ll see in the next article, the path ahead for decarbonizing how we make things is not as clear. Major breakthroughs are still needed to reach a net-zero emissions future.
researchgate.net/figure/Learning-curve-for-new-technologies_fig1_272380872
breakthroughenergy.org/our-challenge/the-grand-challenges
ourworldindata.org/grapher/nuclear-renewables-electricity?country=~OWID_WRL
ourworldindata.org/electricity-mix
Gates, Bill. How to Avoid a Climate Disaster (p. 47).
http://www.renewable-energysources.com/
terrapower.com/doe-natrium-demonstration-award/
news.mit.edu/2019/progress-practical-fusion-energy-0124
Hawken, Paul. Drawdown (p. 6)
renewable-energysources.com
Gates, Bill. How to Avoid a Climate Disaster (p. 141)
youtu.be/1USbcyHHLO0?t=1454
Kai Peter Birke. Modern Battery Engineering: A Comprehensive Introduction (Chapter. 1)
quantumscape.com
twitter.com/elonmusk/status/1365055830085763081
world-nuclear.org/information-library/current-and-future-generation/electricity-and-energy-storage.aspx
irena.org/-/media/Files/IRENA/Agency/Publication/2019/Sep/IRENA_Utility-scale-batteries_2019.pdf
youtu.be/1USbcyHHLO0?t=1726 // https://www.linkedin.com/pulse/energy-storage-technologies-part-iii-mobile-concrete-blocks-kuebler/
heliogen.com/
ourworldindata.org/grapher/share-electricity-fossil-fuels?tab=table // irena.org/newsroom/pressreleases/2021/Apr/World-Adds-Record-New-Renewable-Energy-Capacity-in-2020
Shah, Jigar. Creating Climate Wealth: Unlocking the Impact Economy (p. 138).